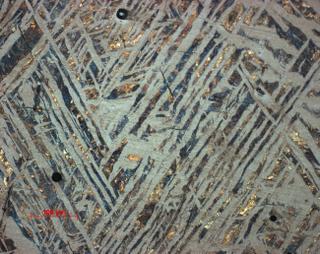
Veas-01 fabricado con Acero tipo Ferrita
En la imágen de abajo, cuyo ancho es de 3.5 milímetros, es posible apreciar la estructura de la Ferrita Widmanstaetten o bandas de desmezcla en
tre carburos y fierro, detectadas en la Roca Veas-01. De acuerdo con los análisis estimados por el profesor Harry Bhadeshia de la Universidad de Cambridge (ver artículo en Blog), las longitudes y anchos de esta ferrita Widmanstaetten son miles de veces mayor que los que se observan en aceros típicos, indicando que el carbono debió haberse reducido en alrededor de un (1) segundo, enfriandose muy rápido este acero, favoreciendo así el desarrollo de esta estructura (ver enlaces con teoría de ferrita aliotromórfica y ferrita Widmanstaetten descritos en el Blog de comentarios de la Universidad de Cambridge).
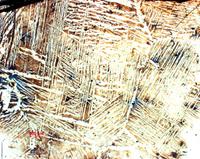
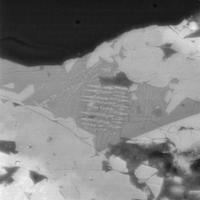
Con respecto al acero de Veas-01, podemos decir, que adicionalmente a las texturas Widmanstaetten, y de acuerdo a los análisis químicos practicados en la USACH y en el IDIEM, el acero presenta un nivel de carbono extremadamente bajo, del orden de 0.03% en peso, lo cual no deja de sorprender si consideramos que la historia de Veas-01 se remonta con anterioridad a 1919, cuando no era posible en Chile ni en el Mundo obtener un grado de pureza en el fierro como el aquí observado.
¿Inclusiones de Sulfuro de Fierro-Manganeso?
Por otro lado, y tal como se observa en la figura de la izquierda, en el interior de este metal existen inclus
iones muy interesantes de Sulfuro de Fierro-Manganeso (Fe,Mn)S, inculsión que no es típica de los aceros y que fue observada a fines de 2004 por el Dr. Bern Lehmann de la Universidad de Clausthal, Alemania. Decimos que se trata de una fase curiosa puesto que el Azufre (S) precipita o con el Manganeso (Mn) o con el Fierro (Fe) en forma separada. En los meteoritos, es común encontrar la Troilita, correspondiente a un sulfuro de fierro, FeS. A diferencia de los meteoritos, en los Aceros de los Altos Hornos se puede apreciar que el azufre precipita con el Manganeso formado el Sulfuro de Manganeso MnS. En nuestro caso, en el acero de Veas-01 se observa que el azufre ha precipitado tanto con el Fierro como con el Manganeso formado esta extraña inclusión.
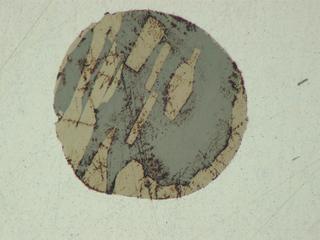
Material denominado Ferrimán o Ferrita
Como si todo esto fuese poco, y además de encontrarse, formando la corteza de Veas-01, abundantes compuestos con estructura espinel (ver informe de Dr. Brian Townley), se observó que el fierro mismo parece poseer estructura espinel, fierro que se denomina ferrita, el cual tiene numerosas aplicaciones tanto en el area de las telecomunicaciones como en la conformación de los discos duros de los computadores.
Como todos bien sabemos, un fierro mientras más puro es, mayor es su conductividad, o lo que es lo mismo, menor es su resistividad. De este modo, para un hierro de alta pureza, su resistividad eléctrica es aproximadamente de 10E(-7) ohm-metros, esto es, un valor inferior a una millonésima de ohm-metro. Al mismo tiempo si a este fierro, que es muy buen conductor, lo sometemos a una disminución progresiva de la temperatura, su resistividad irá disminuyendo también progresivamente, hasta obtener el caso extremo de los conocidos Superconductores, en donde la resistividad es casi cero, debido a la formación de los pares de Copper, y gobernados por la Ecuaciones de London. En otras palabras, en un material conductor la resistividad varia en forma directa con la temperatura.
Lo curioso del acero Veas-01, es que a pesar de tener un alto grado de pureza en fierro de casi 98% en peso, su resistividad es increiblmente alta, y ésta además varía en forma inversa con la temperatura, tal como lo hace una ferrita que hoy fabrica la prestigiosa empresa Ferroxcube.
En este sitio, y si usted está interesado, es posible descargar excelentes y muy explicativos documentos donde se exponen las bases físicas para el diseño y aplicaciones de la ferrita en telecomunicaciones para evitar los típicos problemas de corrientes parásitas que se originan con meteriales conductores.
Resistividad variando en forma Inversa con la Temperatura.
Para el caso de Veas-01 se encontró que durante el día, a una temperatura de 25ºC, el valor de su resistividad se aproximaba al valor de 0.58 (ohm-m), aumentando progresivamente este valor hasta alcanzar los 1.17 (ohm-m), a una tempratura aproximada de 5ºC, durante la noche.
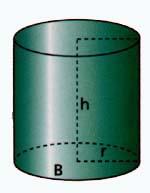
Con respecto al momento magnético de Veas-01, éste es distinto
de cero, aunque los espines son antiparalelos. Con un magnetómetro, facilitado por la Universidad de Chile, se pudo comprobar que los vectores magnéticos emergentes de la piedra no corresponden a la conformación de espines que debiera darse en un fierro o acero, o producto de una colada de fundición, no coincidiendo tampoco la intensidad de los vectores, cuyos valores son mucho mayores que los propios del campo magnético terrestre, tal como lo muestra la siguiente figura 3-D. En esta magnetometría se presenta en rojo las intensidades del campo magnético terrestre, en tanto en verde las propias de la Roca Veas-01, medidas en su superficie.
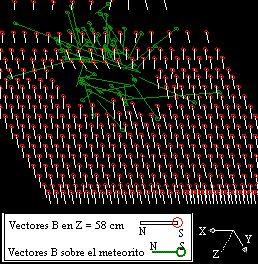
Por último y para dar una breve información sobre a lo que ferritas se refiere, copiaremos textualmente lo que exponen los investigadores John R. Reitz (de la Ford Motor Company) y Frederick J. Milford (Battelle Memorial Institute) respecto al tema:
Ferrita
Según la teoría del ferromagnetismo de Heisenberg, existe un cambio en la energía electrostática relacionada con el cambio de alineación del espín, de paralela a antiparalela, en los átomos vecinos. Si este cambio de energía favorece la alineación paralela y al mismo tiempo es de suficiente magnitud, el material formado por estos átomos es ferromagnético. Si el cambio de energía favorece a la alineación antiparalela, todavía es posible obtener una estructura de espín ordenada, pero con espines que alternan de un átomo a otro a medida que se recorre en cristal.
Una estructura de espín ordenada con momento magnético neto cero se llama antiferroimán. La estructura de espín ordenada más general contiene tanto componentes “de espín hacia arriba” como “de espín hacia abajo”, pero tiene un momento magnético distinto de cero en uno de estos sen
tidos; dicho material se llama ferrimán o simplemente ferrita. Las ferritas más sencillas de interés magnético son los óxidos representados por la siguiente fórmula: MOFe2O3, donde M es un ion metálico divalente, tal como Co, Ni. Mn, Cu, Mg, Zn, Cd o hierro divalente. Estas ferritas cristalizan en una estructura cristalina bastante complicada llamada estructura espinel, como se muestra en la imágen. Así, el ejemplo clásico de una ferrita es la magnetita mineral Fe3O4, que se ha conocido desde épocas antiguas.
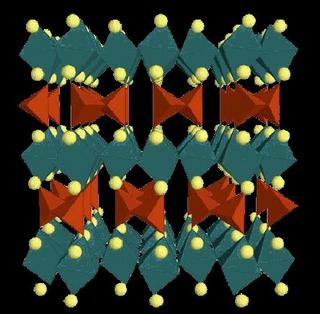
Las ferritas son de considerable importancia técnica porque, además de su magnetización de saturación relativamente grande, son malos conductores de electricidad. Por tanto, se pueden usar para aplicaciones de alta frecuencia en las que las pérdidas por corrientes parásitas en materiales conductores originan problemas serios. Las resistividades típicas de las ferritas están en el intervalo de 0.1 (ohm-m) hasta 10E(4) (ohm-m). En comparación, la resistividad eléctrica del hierro es aproximadamente de 10 E(-7) ohm-metros.